Science
Peter Higgs (1929–2024): A Gentle Giant of Science
We have lost an important scientist; we have also lost a wonderful man.
· 7 min read
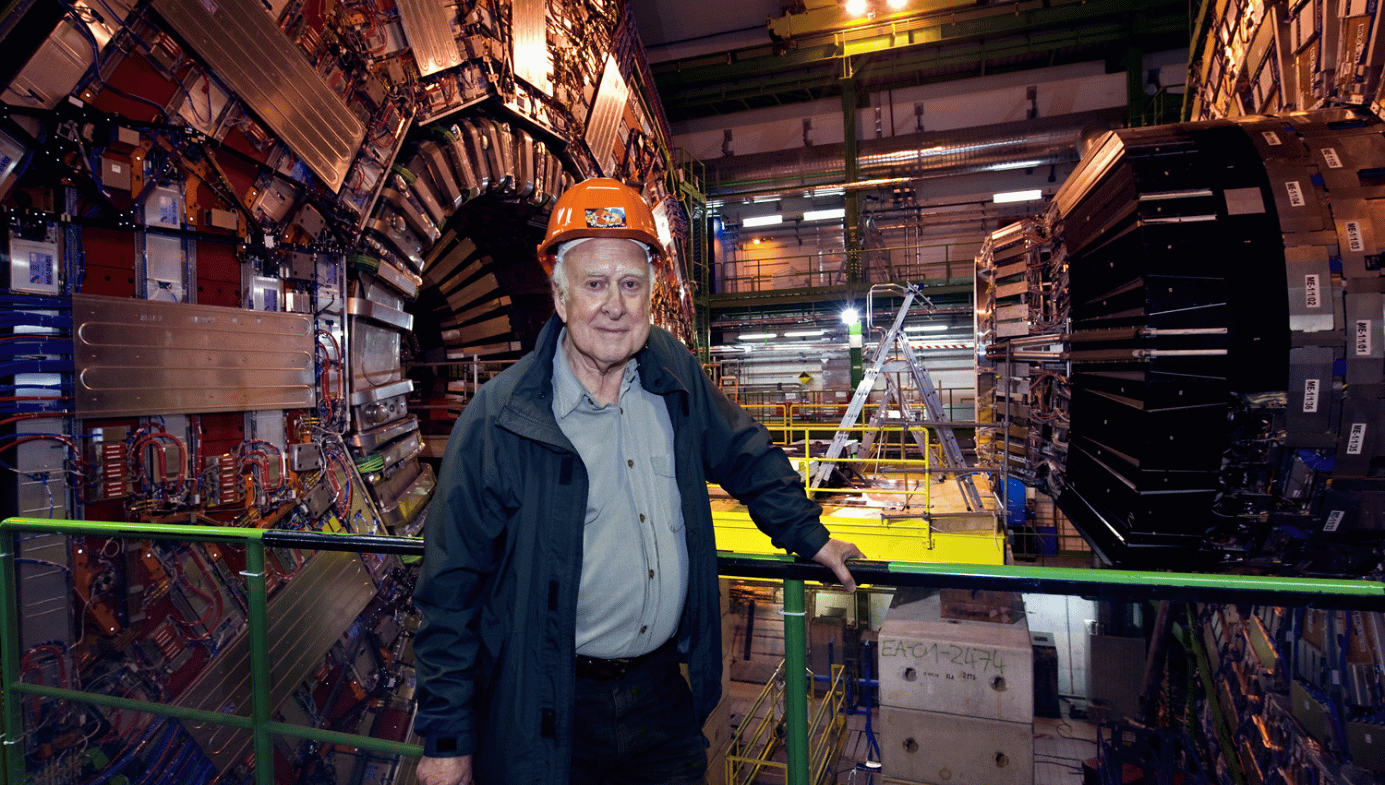
Keep reading
Glamourising Violence at Glastonbury
John Aziz
· 8 min read
From Welfare to Warfare
John Lloyd
· 7 min read
Stealing Australia and Buying New Zealand
Sean Welsh
· 16 min read
After Liberal Internationalism
Matt Johnson
· 21 min read