Fusion Power is Coming
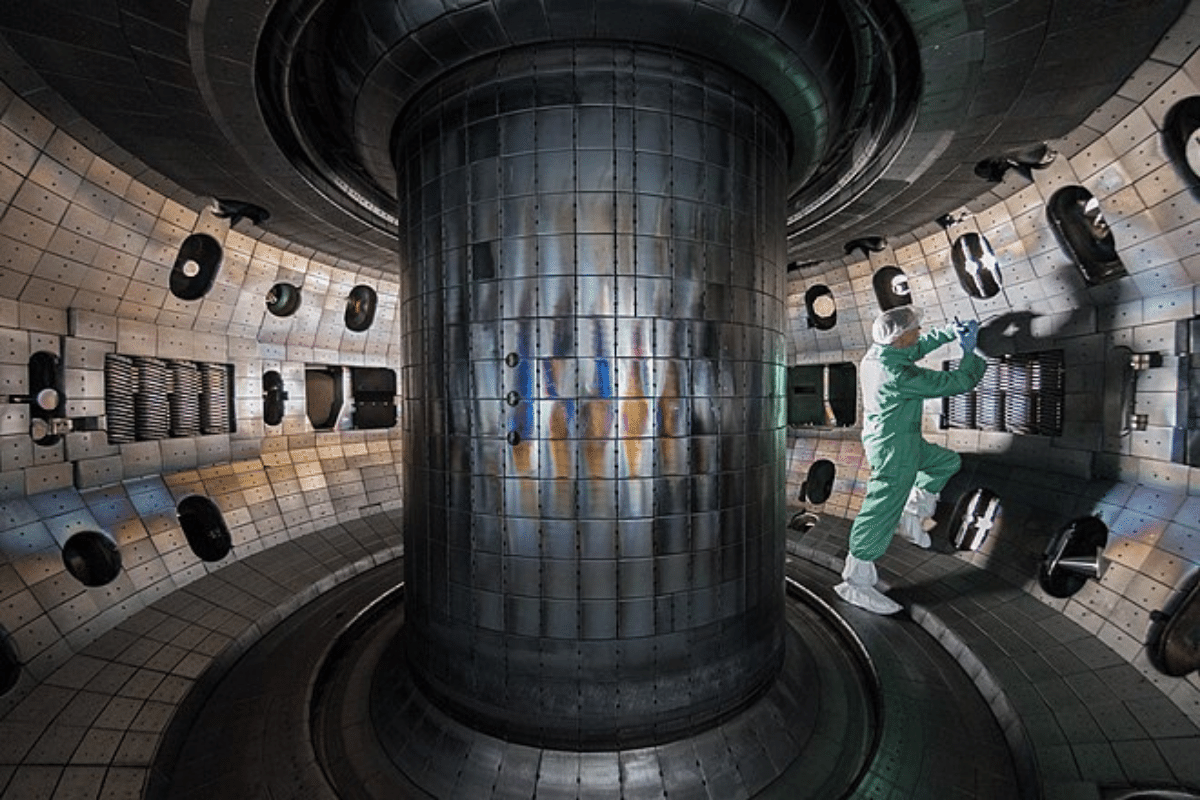
On February 8th, scientists associated with the Joint European Torus (JET) fusion experimental facility located in Oxfordshire, UK, announced that they had achieved a sustained fusion reaction releasing 11 megawatts of thermonuclear fusion power burning a deuterium-tritium plasma mixture continuously for five seconds.
Nuclear fusion energy major breakthrough, created a record breaking 150 million Celsius, 10 times hotter than the Sun for 5 seconds!
— Alvin Foo (@alvinfoo) February 11, 2022
Getting it right will create a disruptive opportunity to power up unlimited energy for eternal. pic.twitter.com/U086oidKPn
The heating power being applied to the plasma was slightly greater than the fusion power released. So while JET did not achieve the milestone of energy “breakeven,” it came very close. The power level of 11 MW was no more than the record that JET, which was built in the 1980s, set in 1997. But this time the burn lasted five times as long.
So three cheers for the JET team. But still, one must ask, why is a tokamak that began operating 35 years ago still the best in the world? Or more broadly, why has the progress developing fusion power been so slow?
It’s a very important question. It was just over a century ago that Sir Arthur Eddington, who had made observations proving the truth of Einstein’s Theory of Relativity in 1919, realized that the mass lost when hydrogen nuclei fuse to form helium must release a stupendous amount of energy in accord with Einstein’s famous equation E = mc2. And that this must be the mysterious source of the power that lights the Sun and all the stars.

Eddington immediately saw the potential practical applications of this discovery. In a speech to the British Association for the Advancement of Science in August 1920 he said: “If, indeed, the subatomic energy in the stars is being freely used to maintain their great furnaces, it seems to bring a little nearer to fulfillment our dream of controlling this latent power for the well-being of the human race—or for its suicide.”
The idea of turning matter into energy remained speculation. That was until Lise Meitner—an Austrian scientist who had fled the expanding Third Reich to Sweden because she was of Jewish heritage—reviewed data sent to her by her former Berlin collaborator Otto Hahn and realized that he had accomplished nuclear fission.

(Meitner, the second woman ever to earn a PhD in physics, was actually a practicing Christian who had served patriotically as an x-ray technician with the Austrian army at the front in WWI, but that cut no ice with the Nazis.) Meitner shared this insight with her nephew, Otto Frisch, when he came to visit her from Denmark for a Christmas party in 1938. Frisch then told his boss, Niels Bohr, just before the latter sailed for New York to meet with Leo Szilard, Enrico Fermi, and a bunch of other refugee and American scientists for a conference. They immediately realized its significance. Before the conference was over, they duplicated Hahn’s experiment, and shortly afterwards, Szilard had his friend, New York financier Alexander Sachs hand carry a letter with Einstein’s signature on it to Franklin Roosevelt to alert the president as to what was now possible.

FDR quickly got the drift. “So, what you are saying, Alex,” he reportedly replied, “is that you don’t want Hitler to blow us up.” Sachs nodded. Thus was born the Manhattan Project.
Nuclear fission works by splitting the heaviest elements into middle weight ones using neutrons as projectiles. It is thus much easier to do than fusion, because neutrons, being neutrally charged, are not repelled by the target nuclei, while making light nuclei fuse requires overcoming the strong mutual repulsion of positive charges. Moreover each fission reaction releases two or three more neutrons, enabling a chain reaction where each fission sets off several more. Thus fission became the basis of the wartime atomic bomb program, and not long afterwards, provided the path to controlled nuclear power, enabling first nuclear submarines in 1954, and then, starting in 1957, commercial nuclear power plants as well.
Yet fusion was not forgotten. Using fission bombs, one can heat and compress a mass of fusion fuel to high enough temperatures and pressures to set it off, with a resulting energy release thousands of times greater than the fission explosion. By the early 1950s, such “hydrogen bomb” weapons were a world-dominating reality. But could not this immense destructive power also be, as Eddington said, put to use for “the well-being of the human race,” instead of just its suicide?
It was clearly worth a try. While fusion of ordinary “light” hydrogen (1H), as occurs in the stars, requires reactors far bigger than any humans can build, the heavy isotopes of hydrogen, deuterium (2H or just “D”) and tritium (3H or “T”) react much faster, making both H-bombs and controlled fusion reactors possible. Deuterium is present on Earth as roughly one out of every 6,000 hydrogen atoms, but even that small fraction packs a big punch. If burned in a fusion reactor, that tiny fraction present in one gallon of any water, fresh or salt, can release as much energy as that obtained by burning 350 gallons of gasoline. For all practical purposes, controlled fusion means infinite energy.
So, starting in 1949 in Britain, and then in the early ’50s in the US and USSR, top secret programs were begun to try to create controlled fusion reactors. Actually, the US and British programs were only secret from each other. The Soviets, through their excellent espionage networks, were well-informed about the progress of both. In fact, the head of the Soviet program, Igor Kurchatov, was better informed about the British program than the Brits themselves were, so much so, that in 1956 he even came to the British Harwell lab to deliver a surprise guest lecture. Without revealing his sources, he then generously explained a mistake that “someone” might make (as the Brits actually were) in measuring the number of fusion reactions occurring within a plasma. (The Soviets apparently wanted the Western controlled fusion programs to succeed, as it would create an energy source they did not have the means to develop themselves.)
Unfortunately, the British didn’t listen, and embarrassed themselves greatly a year later, when, in response to Sputnik, they declassified their fusion program with an accompanying announcement of successful results that proved to be fiction. On the positive side, however, the British announcement led to the declassification of all the controlled fusion programs. This was followed by the wonderful Atoms for Peace conference held in Switzerland in 1958, where the all-male contingent of American fusion researchers had the pleasure of spending many long hours chatting with the vivacious young women of the Soviet translation service, all of whom evidenced an intense interest in experimental and theoretical physics rarely encountered among college girls back home.
Even with declassification however, the Soviets remained the best informed of all the fusion players. So knowing fully the flaws in all the diverse Western approaches, they were able to devise a better one. This was the “tokamak,” a name deriving from the descriptive Russian acronym for “toroidal chamber magnetic.”

It would require getting too technical here to explain why the doughnut-shaped tokamak worked much better than the magnetic mirrors, Z-pinches, theta-pinches, and stellarators being pursued in the West. Suffice to say that it did. By the mid-1960s, the tokamak’s Soviet champion, Lev Artsimovich, was announcing results that were so much better than those achieved elsewhere that no one believed him. So he did the unthinkable. He invited a team of British scientists to come to Moscow and observe and measure the results of experiments on his T-3 tokamak using their own instruments. In 1968, they did so, and confirmed that all of Artsimovich’s claims were true. Tokamak fever swept the West. Meanwhile, the Soviets continued their program, advancing from the seminal T-3 through a series of ever larger machines including T-4 in 1969, T-10 in 1975, and the superconducting T-15 in 1988.

As a result of the tokamak fever, programs supporting alternative approaches to fusion, including not only stellarators, mirrors, toroidal Z-pinches, but novel ideas taking advantage of the self-organizing properties of plasmas, such as spheromaks and field reversed configurations were starved for funds. While few stellarator fanatics continued to hang on in Germany, the last major old guard competitor to the tokamak was the magnetic mirror. But after spending over $200 million to complete the MFTF-B flagship magnetic mirror at Livermore, in 1985 the Department of Energy scandalously cancelled the program before the machine could even be turned on.
But if the fusion program was dangerously narrowed to one major concept, that approach—the tokamak—advanced vigorously.
Driven by spirited international competition, and with a workable approach in hand, over the next three decades the actual amounts of fusion power released in the experimental tokamaks grew a trillionfold.

However, this victorious march towards controlled fusion was halted dead in its tracks when the bureaucrats controlling the major fusion programs got together in the mid-1980s and decided that such competition was wasteful and stressful. Wouldn’t it be better, they reasoned, if instead of competing with each other to build more powerful tokamaks, we all got together on a single big machine? They decided it would be, and called it the International Fusion Test Reactor, or ITER.

Fusion had made steady progress from the ’50s through the mid-’80s driven by a lively competition between the US, European, Soviet, and Japanese programs. But by collapsing these competing programs into a single united effort, ITER, this competitive drive vanished. The result was to bring progress in fusion to a screeching halt, as no new machines were built. Instead, practically all advanced non-tokamak concept research was shut down, and funds that should have gone to build the next generation of tokamaks were diverted to sending high-level bureaucrats to an unending series of summits in Vienna and Kyoto and other lavish places around the world. The ITER design was frozen in an early gargantuan concept, and the program then proceeded at a snail’s pace, with agreement on where to even put the machine not reached for two decades. At this writing the machine is still not built. If it proceeds according to the current schedule, it will not be turned on until 2025, and not attempt to achieve ignition until 2035.
This absurdly glacial rate of advance has caused many people in and around the technical community to become cynical. “Fusion is the energy of the future, and always will be,” became a common quip. But there is reason for hope.
The meetings to plan the ITER program began in the early ’80s, and by the summer of 1985 it was already viewed as a “scandal” by many working in the front lines of the fusion program. At that time I was part of an engineering team at Los Alamos National Lab working on the first design of a fusion reactor based on the then very advanced Spherical Tokamak, or ST, concept. At a group lunch towards the end of the effort, the team leader, Robert Krakowski, reflected philosophically.
“You know,” Krakowski said. “When fusion power is finally developed, it won’t be at a place like Los Alamos or Livermore. It will be done by a couple of crackpots working in a garage.”
We all laughed at that, knowing full-well how the formidable difficulties associated with fusion power development put such a feat well-beyond the capabilities of garage inventors. But in recent years the trend has moved forcefully towards validating Krakowski’s prophesy.
Though the national programs are a shadow of their former selves, and ITER continues to move ahead at the speed of continental drift, something else is going on.
A breakthrough has happened. Through its dramatic and rapid development of reusable launch rockets, Elon Musk’s SpaceX company demonstrated that it is possible for a well-run lean and creative entrepreneurial organization to achieve things—and do so much faster—that were previously thought to require the efforts of major power governments. This has hit observers of the fusion program like a bolt from the blue. Could it be that the seemingly insurmountable barriers to the achievement of controlled fusion—like the barriers to the attainment of cheap space launch—were not really technical, but institutional? Venturesome investors suddenly became interested. Around the world, well-funded entrepreneurial efforts have been launched to make fusion power a reality, and are moving ahead at a pace far outmatching the official government programs. The way things are going, there is an excellent chance that the first controlled thermonuclear fusion reactors will be ignited before this decade is out. Perhaps not by a couple of crackpots in a garage, but by a team of startup company engineers working in a warehouse.
As a result, a whole raft of innovative private fusion power startups are now getting funded (see below).
Fusion is an unlimited source of energy, but there is an even greater power in the universe: human creativity. Fusion will give us wealth. Freedom will give us fusion.
Tokamak Energy
This Oxfordshire England venture, started in 2009 by former staffers from the Culham Laboratory Jonathan Carling, David Kingham, and Michael Graznevitch, has raised $50 million of mostly private money to try to develop the ST (the same concept that I worked on in the 1980s, which was too innovative for ITER to adopt) into a commercial reactor. In a magnetic confinement fusion reactor, the amount of power that can be generated rises in proportion to β2B4, where β is the ratio of the plasma pressure to the magnetic pressure, and B is the magnetic field strength. An ordinary tokamak like ITER can only achieve a β of about 0.12, but an ST can achieve a β of 0.4. As a result, an ST can produce the same amount of power as a regular tokamak in a machine less than 1/10th the size and cost.
Commonwealth Fusion Systems
Founded in 2018, this MIT-based venture has raised $75 million so far, including $50 million from the Italian oil company ENI and about $25 million from the Breakthrough Energy Ventures fund backed by Bill Gates, Jeff Bezos, Jack Ma, Mukesh Ambani, and Richard Branson. The root of the CFS design concept goes back to the 1980s, when the very creative maverick MIT physicist Bruno Coppi proposed achieving fusion in a very small tokamak by the simple expedient of using ultra-strong magnetic fields. The magnetic field lines of a tokamak confine particles to follow them, spiraling around the chamber, with the radius of the spirals being inversely proportional to the strength of the magnetic field. Coppi reasoned that the relevant dimension of a tokamak was not its size per se, but the ratio of its size to the radius of the spiral, because it is this ratio that determines how long a particle will last before it hits the wall. Furthermore, as noted above, the higher the magnetic field strength, the faster the particle is likely to react. So if you want a particle to take part in a fusion reaction before it hits the wall (which would cool it too much for fusion), the key is just to go for broke with ultra-powerful magnets. But the problem is that the highest magnetic field it is practical to achieve with traditional low temperature superconducting magnets is about 6 Tesla, and Coppi needed 12 T. So, he designed an experimental machine called “Ignitor” using copper magnets. This could not be a practical commercial reactor, because the resistive copper magnets would use too much power. Nevertheless, if it had been built, we probably would have achieved thermonuclear fusion ignition in the 1990s. But all of the US Department of Energy funds were committed to ITER, so Ignitor was never built. But starting around 2014, an MIT group led by Professor Dennis Whyte decided to pick up where Coppi had left off, improving on the Ignitor concept by making use of high temperature super conductor magnets, which require no electric power and can reach 12 T. As a result, with more than twice the magnetic field strength as ITER, the CFS reactor, known as SPARC (for Smallest Possible Affordable Robust Compact) fusion reactor, will achieve 1/5th the power hoped for by ITER in a reactor 1/65th the volume. Furthermore, CFS aims to do it by 2025, achieving in seven years what ITER hopes to do in half a century.
Tri Alpha Energy
Founded in 1998 by the late Dr. Norman Rostoker, southern California-based TAE recently received over $800 million in investment from heavy hitters including Microsoft Co-founder Paul Allen, Goldman Sachs, Wellcome Trust, Silicon Valley’s NEA, and Venrock. TAE’s departure from orthodoxy is more radical than the above mentioned startup in that they do not use a tokamak or toroidal chamber of any kind. Instead, TAE uses a simple cylinder chamber, with the required toroidal magnetic field induced in the plasma itself by having a linear magnetic field created by an outside solenoid suddenly reversed, causing it to curve around and connect to itself. This creates a kind of smoke ring current vortex in the plasma, or what is called in the fusion business a “field reversed configuration” or FRC. When I was in graduate school in the 1980s at the University of Washington, FRCs were all the rage, as they routinely achieve β values over 0.5. Moreover their simple cylindrical construction makes them potentially much more promising for creating low cost commercial systems or fusion rocket drives than tokamaks. But by the 1980s, tokamaks had crowded out all funding within with US fusion budget, and shortly afterwards even the US tokamaks were starved from funds to feed ITER. FRCs were far too avant-garde to even be considered by ITER. But private investors are much more daring than international bureaucrats, and TAE is pushing hard, with a goal of demonstrating net energy production by 2024.
Helion Energy
Founded in 2013 by Dr. David Kirtley, Prof. John Slough, Chris Pihl, and Dr. George Votroubek, Helion uses two FRCs that are accelerated into a cylindrical reaction chamber from opposite ends to collide in the middle where they are compressed by a solenoidal magnetic field to reaction conditions. Fusion reactions then heat the FRC plasma, causing it to expand back towards the chamber ends at high speed, with its energy being directly converted into electricity in the process. The cycle would then be repeated once per second to keep making power, or alternatively rocket thrust. In November 2021, Helion Energy announced the close of its $0.5 billion Series E, with an additional $1.7 billion of commitments tied to specific milestones. The round was led by Sam Altman, CEO of OpenAI and former president of Y Combinator. Existing investors, including co-founder of Facebook Dustin Moskovitz, Peter Thiel’s Mithril Capital and the Capricorn Investment Group also participated in the round.
General Fusion
Founded in Burnaby British Columbia by Dr. Michel Laberge and Michael Delage in 2002, GF has since received some $130 million in investment. The GF concept injects an FRC into a chamber containing a rotating liquid metal wall, which is then driven inward by an array of pistons to compress the FRC to fusion conditions. This is a variant of the “imploding liner” concept that has a heritage going back to the AEC’s 1972 Project LINUS. The theory behind it is complicated, but appears sound. GF hopes to show it will all work in the mid-2020s.
Lockheed Martin
In 2010, under the inspiration of Dr. Tom McGuire and Charles Chase, Lockheed Martin initiated its own “Compact Fusion Reactor” development program using internal funds. The CFR appear to be a linear cylindrical system, confined at the ends by increased magnetic fields (or “magnetic mirrors”) but with an extra pair of superconducting magnetic coils operating inside the plasma chamber to make “cusps” that improve confinement. This creates a very attractive magnetic field configuration, but the engineering to make it work in an actual thermonuclear system seems quite challenging.
EMCC
In 1987, the late visionary Robert Bussard (of Bussard ramjet fame) revived a 1950s concept originated by Philo Farnworth (the inventor of television) to use electrostatic fields, rather than magnetic fields, to confine a fusion plasma. The idea works well enough that a very simple system can be used to generate a lot of fusion reactions, as demonstrated by neutron production, but all sorts of bells and whistles, including auxiliary magnetic fields are needed to get it close to generating net power. Bussard managed to get preliminary funding from the US Navy but now he is gone, and the rest of the team, led by Dr. Paul Sieck and Dr. Jaeyoung Park are seeking private funding. Any takers?
Others
In addition to the above, there are quite of few dark horses in the race. These include New Jersey based Lawrenceville Plasma Physics Fusion, led by Dr. Eric Lerner, who has produced interesting results using a concept called the plasma focus; CT Fusion, a University of Washington based project founded by Dr. Tom Jarboe, Dr. Aaron Hossack, and Derek Sutherland, which is pursuing an FRC-like approach known as a “spheromak”; Applied Fusion Systems, founded in 2015 by Richard Dinan and Dr. James Lambert, who are trying their luck with an ST; Helicity Space, a company founded by Setthivone You, Marta Calvo, and Staphane Lintner, which is developing Dr. You’s concept of taking advantage of the self-organizing plasma process that creates solar prominences to develop fusion reactors and fusion rockets; the Australian HB11 Energy company, which is applying Heinrich Hora’s unique combined laser-magnetic fusion concept to try to ignite the difficult p-11B fusion reaction; and Hyper V, NumerEx and the Sandia Lab/University of Rochester-based MagLIF project, which are all attempting to develop variants of the imploding liner concept.
CORRECTION: An earlier version of this article mistakenly stated that Helion was founded in 2005 by Prof. John Slough alone. This section has been amended. Apologies for the error.