Where Did We Come From? An Astonishing Hypothesis
The possibility that non-life became life over a million or so years, rather than hundreds of millions of years, is astonishing.
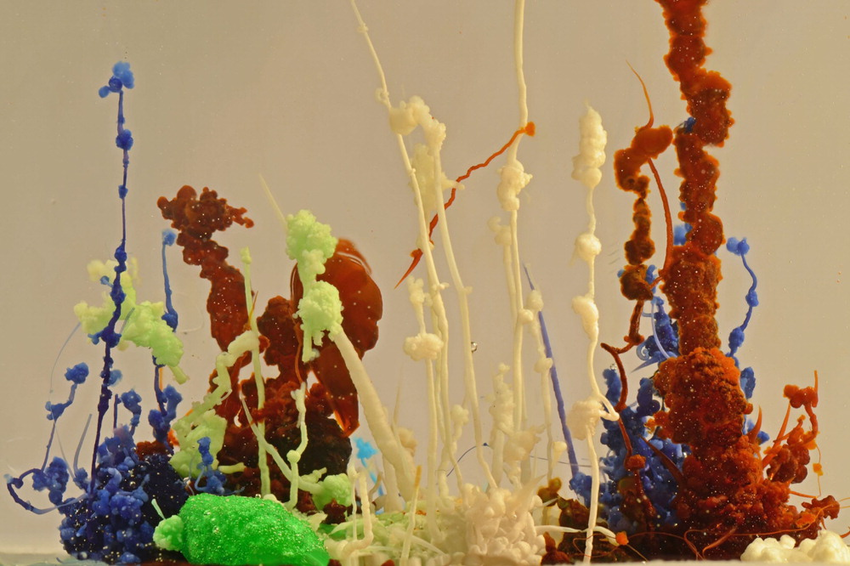
“Where did we come from?”
Nick Lane’s The Vital Question: Energy, Evolution and the Origins of Complex Life tries to answer this question. He looks back to two moments in time:
1. When bacteria/archaea appeared on Earth about 4 billion years ago.
2. When complex life (eukaryotes) appeared about 2 to 1.5 billion years ago.
Lane discusses both of these events. This article will restrict itself to Lane’s hypothesis about the first of these events: the likely emergence of simple cellular life (bacteria and archaea) from an alkaline hydrothermal vent located near a mid-ocean ridge.
I shall also discuss recent research about the likely evolution of viruses. This indicates that the ancestors of modern viruses were independently replicating virocells that co-evolved with the bacteria and archaea, and all three of them – virocells, bacteria and archaea – had a last universal common ancestor (LUCA).
Lane’s book does not have a detailed discussion of viruses. Nor does the virocell literature discuss where virocells may have evolved. But if Lane and virocell theory are both right, the first living cells emerged from an alkaline hydrothermal vent field where they had evolved over the lifetime of the vent field – say one million years – in a cellular Garden of Eden!
For non-scientists like me it is not hard to understand how life evolved from simpler to more complex forms. The evidence is in rocks and there is a simple explanation: survival of the fittest. But we become less confident when we get to the start date about 4 billion years ago.
How did a mixture of rock, liquids and gas come alive? The rocks tell us very little, and prebiotic chemistry and even the simplest cellelular machinery are awfully complicated. The possibility that non-life became life over a million or so years, rather than hundreds of millions of years, is astonishing.
The American botanist, Bill Martin, who is head of the Institute for Molecular Evolution at Heinrich Heine University, Düsseldorf, has written:
One might ask: Is it important for evolutionary biology to provide a better understanding of the very earliest history of life? It is arguably one of the most important frontiers facing science, specifically as evolutionary biology interfaces with society. One might also ask: Can we ever understand anything as complex as the origin of life and early evolution? The answer is unquestionably yes, the issue is merely when we will attain that understanding.
One of the theories is that life got started with RNA – the “RNA World” theory, which says RNA existed before DNA and proteins. All living cells have both RNA and DNA. RNA has four nitrogenous bases (adenine, guanine, cytosine and uracil), repeated in a single strand, unlike DNA’s double stranded helix. DNA has RNA’s first three nitrogenous bases, but it substitutes thymine for RNA’s uracil. The acronyms A, G, C, U and T are ubiquitous in diagrams showing the structure of RNA and DNA molecules. Although RNA is less stable and more reactive than DNA, it is a simpler molecule for the start of life.
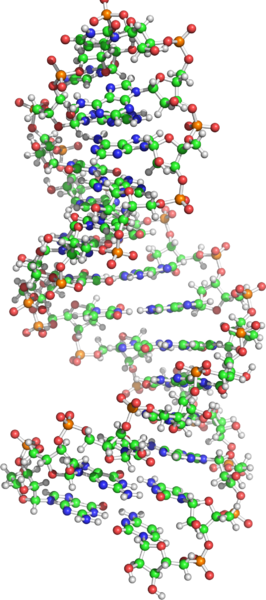
Readers of popular science are likely to be familiar with the 1953 “primordial soup” experiment of Stanley Miller and Harold Urey where electricity was discharged through flasks of water and what were believed to be the gases in the early Earth’s atmosphere. Miller and Urey synthesised amino acids which are the building blocks for life. The electricity discharges in the laboratory had a role equivalent to lightning in the ancient atmosphere. As Lane says: “Suddenly the origin of life looked easy!”
This was a comforting answer to biology’s most awkward question. Miller and Urey conjured up a nourishing alphabet soup of molecules. With a little bit of electricity, the molecules became more complicated, swapping and combining letters like geeks exchanging tips about computer games. It was possible to imagine that in the ancient oceans longer and longer strings of molecules continued assembling and then hey presto! Infant bits of meaningful RNA or DNA started replicating.
Darwinian competition is absent from this model. The bits of information did not have to think about making a living. They were sustained by lightning discharging through this friendly brew of active molecules.
It was made to sound easy. But 56 years went by before researchers were able to get a ribonucleotide (one of the building blocks of RNA) to assemble itself from simple precursor chemicals in a laboratory. John Sutherland and his colleagues from the University of Manchester tried for 12 years to get this to happen. Then in 2009 they announced in Nature they had created a ribonucleotide, a building block of RNA, from simple chemicals under conditions that might have existed on the early Earth, using what they called “molecular choreography”, where the molecules were their own choreographers. Sutherland’s team synthesised cytosine and uracil (C and U), nitrogenous bases known as pyrimidines, but not the purines guanine or adenine (G or A).
It was still unclear how the large purine bases adenosine and guanine might have arisen on prebiotic Earth. Then in 2016 (more than 60 years after Miller and Urey’s primordial soup experiment) it was announced in Science that a similar synthesis in the laboratory of the purine bases had been achieved by Sidney Becker et al. The journal’s editors explained: “the A and G bases can be synthesized easily and in high yield from prebiotically reasonable precursors, lending further support to the RNA World hypothesis.”
Steven Benner of the Foundation for Applied Molecular Evolution in Alachua, Florida has pointed out that the chemical conditions that gave rise to the purines produced by Becker et al. do not match the conditions that Sutherland’s group suggested may have led to the pyrimidines. Benner says it is still unclear how As and Gs (the purines) linked up with the Cs and Us (the pyrimidines) to form RNAs.
The late Robert Shapiro, a chemist at New York University, was critical of RNA World theories. He said: “The flaw with this kind of research is not in the chemistry. The flaw is in the logic – that this experimental control by researchers in a modern laboratory could have been available on the early Earth.” He was of the view that RNA was too complex to have emerged from small molecules. He believed simpler metabolic processes eventually catalysed the formation of RNA and DNA.
Nick Lane is a biochemist who is actively carrying on research in the field he is writing about. He points out Earth’s early atmosphere was probably more like our own minus oxygen, and not the Hadean mixture used in Miller and Urey’s primordial soup. Nor, in his view, were Miller and Urey’s electricity discharges in the laboratory equivalent to lightning in Earth’s ancient atmosphere. Lane once calculated that to sustain a tiny primitive biosphere, such as the biosphere that existed before photosynthesis “by lightning alone, would require four bolts of lightning per second, for every square kilometre of ocean . . . There are just not all that many electrons in each bolt of lightning.”
Lane criticises the significance of Sutherland’s findings. Sutherland’s “plausible primordial conditions” rely on UV (ultraviolet) radiation and cyanide (HCN, a gas that smells like almonds). Lane writes: “No life on earth uses cyanide as a source of carbon; and no known life uses UV radiation as a source of energy. Quite the contrary, both are considered dangerous killers.” What’s more, Lane points out, cyanide would most likely have been present in concentrations that were too small to be a reliable carbon source.
The sharing of certain universal genes by all living cells indicates there is a last universal common ancestor of all life. A recent study led by Bill Martin has found LUCA probably had 355 genes – a number that was “flabbergasting” to him. By way of comparison humans have about 20,000 genes. LUCA was already complex when it formed.
Lane says six basic properties are shared by all living cells:
- A continuous supply of reactive carbon to synthesise new organics;
- Free energy to drive the formation of new proteins, DNA, and so on;
- Catalysts to speed up and channel these reactions;
- The excretion of waste;
- A cell-like structure (or “compartmentalisation”) to separate the inside from the outside;
- DNA, RNA or its equivalent to specify the inheritance of form and function.
The sudden and perhaps almost simultaneous acquisition by replicating amino acids of all six of these traits on the ancient Earth seems little short of miraculous. Researchers had to discover an environment where the physical setting performed some of these functions on behalf of early predecessors of LUCA, allowing incremental development.
Polymers are large molecules composed of repeated subunits and include plastics and DNA. To drive the polymerisation of long chain molecules such as DNA requires the removal of water for each new bond that is formed. How this could happen in a watery environment, where life appears to have evolved, was a mind-numbing distraction for some researchers. As Lane suggests, it was “a bit like trying to wring out a wet cloth under water”. The answer to this problem was a continuous supply of organic carbon and something resembling the ATP molecule (such as acetyl phosphate which could have been formed by early geologic processes), that takes up a molecule of water when it is split.
To build a cell from scratch, as Lane puts it, needed a “high flux of carbon and energy that is physically channelled over inorganic catalysts”. Naturally occurring inorganic complexes, such as metal sulphides, could have been the ancient catalysts and are still conserved in certain proteins that are universal to life. Darwin’s warm ponds and the primordial soup were not candidates for the environment where the predecessors of LUCA could develop. But hydrothermal vents were.
Submarine black smoker vents were discovered in 1979 and hailed as a candidate. They form along mid-ocean ridges where the tectonic plates of continents are moving apart and water penetrates fissures and interacts with volcanic magma. These vents would have been different 4 billion years ago when there was no free oxygen. They would have been highly acidic and very hot – too extreme for organic chemicals to remain stable.
Lane explains that in 1988 Mike Russell, a geochemist, suggested warm alkaline springs at the bottom of the acidic Hadean ocean could have provided the environment in which life began. Modern equivalents of the warm alkaline springs predicted by Russell were not discovered until 2000 when a submarine hydrothermal vent (which was called “Lost City”) was found about 10 miles from the mid-Atlantic ridge, matching almost all of Russell’s predictions.
The photographs below compare an akaline hydrothermal vent at “Lost City” (A) with a submarine black smoker vent (B). The alkaline vent is 60 metres high, about three times the height of the black smoker vent. Unlike the black smoker, the alkaline vent is not above a magma chamber, and is some miles away. Lane says alkaline vents “are not superheated, but warm, with temperatures of 60 to 90 degrees Celsius . . . riddled with a labyrinth of interconnected micropores.” These vents form when water penetrates through fissures in the ocean floor near mid-ocean ridges, then rises through rocks rich with minerals such as olivine, and reacts with the olivine to create the hydrated mineral serpentinite, which has a mottled green appearance. This reaction (serpentinisation) generates large amounts of hydrogen gas dissolved in warm alkaline fluids. 4 billion years ago these fluids met with ocean water which was rich with dissolved trace elements necessary for life and was acidic. (Dissolved salt was yet to make the ocean alkaline.) The result was a “flow reactor”.
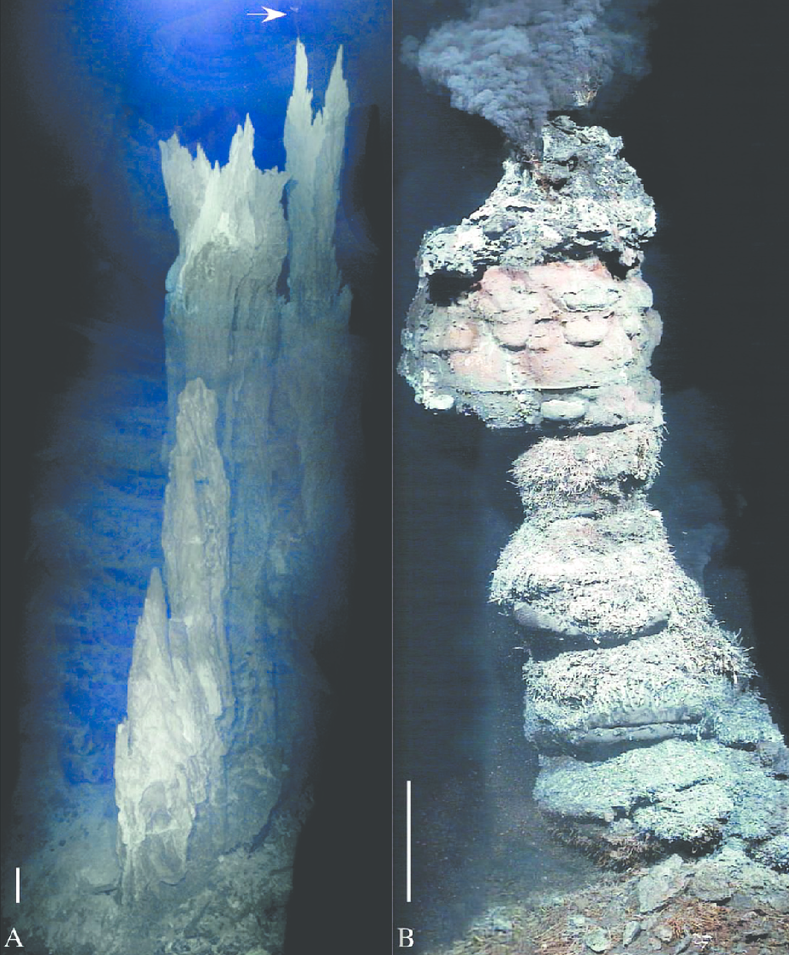
Mike Russell has written about how he formed his astonishing hypothesis. One evening he was entertaining his son Andy with some “chemical gardens”, a kit available from a toyshop. Hydrated crystals of a strong acid and weak base (such as cobalt chloride) are immersed in an alkaline solution of sodium silicate. Long colourful fingers of silica then form. Next night Andy began breaking them up. Deaf to pleas to join his family for supper, Andy called out from behind a locked bathroom door, “Hey Dad, these things are hollow!” This was Russell’s Eureka moment.
Hollow! Suddenly I understood the puzzling mineral patterns I had seen in 350-million-year-old rocks from a lead-zinc mine in Ireland. Those columns, chimneys and bubbles in the stone were made of iron sulfide – they must have once been natural chemical gardens.
He consulted with a colleague Allan Hall next day and they realised:
such structures could have acted as an inorganic membrane for the first cells when warm, alkaline-spring water rich with sulfide ions met acidic seawater laced with iron from black smoker vents. We rushed to the laboratory and readily mimicked the fossil shapes by simulating these conditions.
As well as providing membranes, the iron sulphides were catalysts for a reaction between the hydrogen generated by serpentinisation and carbon dioxide dissolved in the ancient ocean (which was at high levels – the atmosphere also had high levels of carbon dioxide). Russell explained:
H2 and CO2 are stable in each other’s presence. Indeed, the fact that hydrogen and carbon dioxide do not fuse spontaneously – despite the chemical potential to do so – is the reason life exists. Life is both the catalyst for and the product of this reaction. Here it is at its simplest:
2H2 + CO2 → [CH2O]proto-life + H2O
Bill Martin teamed up with Russell in several papers. In 2004 Martin and Russell drew attention to the close similarity between iron-sulphur minerals at vents and iron-sulphur clusters embedded in modern enzymes.
Alkaline hydrothermal vents therefore supplied the chemistry kit and the environment – a flow reactor made up of micropores – that may have allowed living cells to get started, step by step internalising processes they duplicated from the abiotic reactions that surrounded them.
In Martin’s recent study to determine LUCA’s probable genetic composition, although he found it may have had a “flabbergasting” 355 genes, his team did not find the genes involved in making amino acids, the building blocks of proteins. He says LUCA may have depended on amino acids produced spontaneously at vents and abiotic reactions to make many of the chemicals it needed. It may have only been able to replicate itself in the sheltered workshop of one of these vents. For this reason Martin has described LUCA as “half-living”.
But eventually fully-alive cells floated free from a vent.
Lane’s book discusses viruses only briefly. Viruses are widely considered to be “not-life” as they cannot replicate independently. However, one might think they are a candidate for the progenitor to living cells. They have less complex genomes than cells and consist of DNA or RNA that is coated in a protein shell (a capsid). The capsid protects the virus’s genome from lethal chemicals, enzymes and extremes of temperature and radiation and allows them to circulate freely outside cells and remain viable.
Lane explains: “In every millilitre of seawater, there are ten times as many viruses, waiting for their moment, as there are bacteria.” Their moment is when they invade a living cell and hijack its metabolism to replicate themselves.
In addition to Lane’s discussion, I wanted to understand more fully how viruses fitted into the picture. Not long before Lane’s book was published two landmark studies were published by a group of three authors about the evolution of viruses: Giant viruses coexisted with the cellular ancestors and represent a distinct supergroup along with superkingdoms Archaea, Bacteria and Eukarya; and Viral evolution Primordial cellular origins and late adaptation to parasitism.
Nasir and his co-authors took advantage of available protein structural and functional data to construct a tree of life for viruses, archaea, bacteria and eukaryotes. Proteins may fold in a particular way because physics and chemistry favour a particular packing arrangement. A fold family is a group of proteins that have similar arrangements because they are related to each other through evolution and there was a common ancestor. A fold superfamily (FSF) is a larger and more diverse fold family where there was a more ancient common ancestor. Nasir et al constructed their tree of life using protein fold superfamilies, because they are less subject to horizontal gene transfer and other problems affecting phylogenomic trees based on genes.
They examined the three possible hypotheses for the evolution of viruses:
- Viruses evolved before cells and were the founders of cellular life.
- Viruses are parasitic reductive products of ancient cellular organisms. They have jettisoned some of their cellular machinery and genome to become parasites.
- They are escapees of modern genomes.
The problem with the first hypothesis is that viruses cannot replicate independently. How could they have founded cellular life when they need the metabolism of cells to replicate? The escape hypothesis fails because of the presence in viruses of unique structures not found in cells.
The evidence from protein families favoured the second hypothesis. Newly discovered giant viruses have large genomes – some have more than 1,000 genes – and have retained a partial encoding translation apparatus that “is most likely the remnant of an advanced functional apparatus that was once present in the ancestor of these viruses”. The presence of this partial encoding translation apparatus, the authors said, “now challenges the boundaries between cells and viruses.” The fold superfamilies shared by giant viruses with cells are ancient.
When did viruses adopt a parasitical life style? The protein molecular clock (molecular variations accumulating over time) indicates that the proteins forming capsids (the coating of viruses that allows them to circulate freely and parasitise cells) evolved as recently as about 1.6 billion years ago – at about the time complex life evolved! This finding contradicted a widely held expert view that capsids had an ancient lineage. Nasir et al. concluded that parasitism in viruses is “an afterthought triggered by genome reduction” and large to medium sized viruses or virocells co-evolved with the ancestors of cells as shown in their tree of life below.
(I am guilty of cherry-picking in this article, by accepting the finding of Nasir et al. that a molecular clock analysis is valid when determining the date when capsids evolved, while being sceptical about its relevance for the origin of life. The correctness of their second hypothesis is critical for the issues discussed here. The date when capsids evolved is not, so long as they evolved after the first cells evolved.)

A universal tree of life of Nasir et al. inferred from a phylogeny of protein domains. Time unfolds from bottom to top according to the age of FSF protein domains (nd) in a relative 0–1 scale and in geological time (billions of years, Gy) according to a molecular clock of folds.The horizontal axis is proportional to the number of FSFs. Extant FSF repertoires are indicated for supergroups (superkingdoms and viruses). The FSFs that are unique to supergroups are highlighted with different color shades. The common ancestor of the lineages of cells and large-to-medium-sized DNA viruses (LUCA) and the common ancestor of cellular organisms belonging to superkingdoms Archaea, Bacteria and Eukarya (LUCELLA) are indicated with circles at the base of the universal “tree of life.” The bar plots show FSFs that are unique to supergroups or that are shared with viruses or cells.
Nasir et al. do not discuss the environment where virocells may have first evolved. The independent metabolism they once may have had, may have given some clues about this. But only vestiges of this have been conserved in a few very large viruses. However their finding that ancient virocells (the ancestors of modern viruses) may have co-evolved with archaea and bacteria does not, in my view, contradict the alkaline hydrothermal vent theory. It may simply add to it. The implication is that LUCA had three immediate progeny, and not two.
When I started writing this article, which began as a review of Lane’s book, I entertained the possibility that some combination of the primordial soup theory and alkaline hydrothermal vents was a possibility. Perhaps there were freely circulating bits of DNA or RNA in the form of viruses, survivors from failures to achieve replicating cells, that hopped from vent to vent, seeding new attempts to achieve independently replicating cells, until finally LUCA gave birth to a cell that could live outside the vent. This combination of soup and vents allowed a leisurely time frame – perhaps many millions of years – for the development of Lane’s six properties of cellular life.
If capsids developed as recently as 1.6 billion years ago, small viruses could not have seeded hydrothermal vents when cellular life was getting started. Lacking a protective protein coat they would have quickly degraded in ancient oceans. Nor is it likely that some early equivalent of plasmids – small circular bits of DNA in cells that are separate from chromosomal DNA – circulated independently. Modern plasmids have no protein coating and hop from cell to cell only when the cells are in physical contact. Lane points out that Earth’s early oceans were hostile environments for the first ancestral cells when (and if) they escaped from vents. With no atmospheric ozone, deadly ultraviolet radiation bombarded their upper layer and they were nutrient-poor compared with the rich brew of chemicals that may have fed LUCA in her cosy vent.

The finding that capsids are likely to have evolved 2 billion years after cellular life began is explosive for the RNA World theory. This theory attractively suggested life began as a self-assembling instruction manual, and the metabolism or motor was then assembled with help from the manual.
Russell and Martin’s vent theory suggests metabolism and genes developed together, sending each other encouraging messages. Our hearts and brains evolved in tandem.
If freely circulating DNA and RNA with no metabolism did not become viable until 1.6 billion years ago and LUCA could only evolve in an alkaline hydrothermal vent, this implies that cellular life had only a short time frame to evolve from non-living to living matter. Prototypes of LUCA could not escape from a vent (or vent field) that was about to become inactive and find a younger, active vent field. Life had to get started in the flow reactor of a particular vent field and develop the ability to survive independently while the vent field was still active. This implies a time frame of perhaps a million or so of years, and not hundreds of millions of years.
Alkaline hydrothermal vents form at the edge of submarine ridges where tectonic plates are separating. The Lost City vent field has been active for more than 30,000 years. The slow continual movement of tectonic plates means that vent fields are continually being replaced. Früh-Green et al. suggest: “ . . . the amount of heat produced by serpentinization has the potential to drive moderate-temperature, Lost City–type systems for hundreds of thousands, if not millions, of years.”
This time frame for non-life to become life may seem to lack credibility, particularly if LUCA had to develop Martin’s “flabbergasting” 355 genes and a fully alive cell had to have even more genes to survive outside the vent field.
A quick glance at the tree of life of Nasir et al. suggests LUCA took two or three hundred million years to develop from its first beginnings. Virocells then broke away. Then another two or three hundred million years went by as LUCA became LUCELLA (ancient ribocells). LUCELLA was also not in a hurry. She dawdled through another hundred million years or so before she split into the superkingdoms of the archaea and bacteria.
Bill Martin’s 2011 article “Early evolution without a tree of life” suggests a tree of life is useful only for complex life (the eukaryotes) and is irrelevant for archaea and bacteria. While some recent relationships can be established by a tree-like structure for archaea and bacteria, the branches disappear further back in time. Lane’s book says fundamental differences in biochemistry and genetics make it clear archaea and bacteria are different domains, but horizontal gene transfer and “perhaps the erosion of a statistically robust signal over an unimaginable 4 billion years of evolution” has resulted in the “amazing disappearing tree”. Lane quotes this term from Martin.
We may also be confronting some definitional issues here. The tree of life of Nasir et al. is a tree of life’s domains. Nasir et al. are tracking the molecular age of certain proteins, some of which are unique to the different domains. They are not trying to track the phylogenic history of individual species of cells, which is Martin’s criticism of trees of life.
There is also a definitional issue with the term LUCA. Martin’s half-alive LUCA, which could not exist outside an alkaline hydrothermal vent, may not be the LUCA referred to by Nasir et al. Nasir et al. seem to be referring to a fully functioning cell.
Before there was a fully-functioning cell there had to be a partly-functional or half-alive cell. It is here that vent theory comes into its own. If we briefly ignore the complex chemistry, there is a simple reason why a vent or some enriched chemical environment must have been the place where life began. In his 2011 disappearing tree of life article Bill Martin explains it in one sentence: “Let’s recall that replication means doubling of mass, hence the precursors for any doubling need to be in steady supply, ideally at their site of synthesis.” Martin argues: “Life is a chemical reaction, not stirred organic soup.”
The molecular clock was developed by comparing molecular change in complex life forms with fossil evidence. Francisco J. Ayala challenged the hypothesis that molecular change proceeds at a steady rate for a particular gene, and concluded that “molecular evolution is dependent on the fickle process of natural selection” although it “often yields an approximate clock”. Simple life forms diversify and adapt quickly. They have less baggage that has to be juggled. The Cambrian explosion starting 541 million years ago lasted for not much longer than 20 million years and almost all modern phyla appeared.
A molecular clock measures molecular change. Is it even relevant when we are trying to determine how long it took to make a gene that previously did not exist? In the flow reactor of the vent field, wild experiments may have occurred in quick succession. Fragile new combinations had to become robust quickly – or they became a dead end. The molecular clock had to tick very rapidly, otherwise it stopped. In this way 500 million years of the molecular clock may conceivably have been compressed into 1 million years.
The molecular clock relies on fossil evidence. There is not much fossil evidence about the early history of life’s domains. But if life got started in an alkaline hydrothermal vent, there is a geological clock we can use as a reference point – the lifespan of a vent field.
There is also another way of viewing a short time-frame during which non-life may have become life. Proto-life may have started in numerous vent fields that failed to produce fully functioning cells. There may have been other vent fields where fully-living cells escaped and died without survivors in the hostile ancient ocean. Proto-life could have flickered uncertainly perhaps for hundreds of millions of years in this way.
Eventually (so it appears) there was a series of lucky events at a particular vent field, the molecular clock ticked very rapidly, and within a million or so years cellular life emerged from there and escaped into the depths of the abyssal ocean, away from ultraviolet radiation, and survived. This is fantastic, if it’s true.
If LUCA was not viable outside a vent, the implication is that living cells escaped from the same vent field in perhaps three different flavours – archaea, bacteria and virocells, which is even more fantastic.